
This Article From Issue
July-August 2016
Volume 104, Number 4
Page 201
DOI: 10.1511/2016.121.201
Batteries have their limitations. Even ubiquitous lithium batteries don’t scale well to small sizes, and they perform poorly in harsh conditions. Michael Spencer (below), an electrical engineer and computer scientist at Cornell University and a Sigma Xi Distinguished Lecturer, works on power generation with relatively low-energy radioactive sources. Spencer explained what these so-called betavoltaic devices are, and why they are now gaining popularity, to managing editor Fenella Saunders. (Spencer also works with twodimensional materials, such as graphene, that are one molecule thick; his comments about how those materials are, and are not, living up to their promised usefulness in electronics are included in the video of this interview)
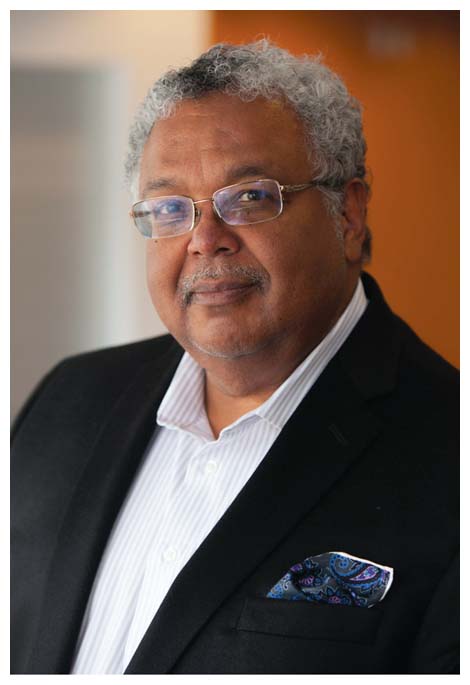
What is a betavoltaic device?
Everybody knows what photovoltaics are; they produce a voltage by absorbing photons. Betavoltaics are a direct analogy to that, but instead of photons, they absorb high-energy electrons that are the product of radioisotope decay. If the radioisotope produces only electrons, it’s called a beta emitter.
What materials release these high-energy electrons?
The most popular source that we use is tritium, an isotope of hydrogen. The tritium is in the gas phase, but to make a device out of it we diffuse the tritium into titanium, where it bonds with the matrix and forms a metal hydride. This metal matrix is the source of the high-energy beta electrons. You place that right against your detector.
How easily can these high-energy particles be shielded?
Beta electrons are stopped very easily. For example, they’d be stopped by just a layer of dead skin; they don’t penetrate very far.
But there are safety limitations imposed by the regulatory agencies about how much radioisotope one is allowed to use in these devices, so you get certain power limitations.
Are there any devices that already use these isotopes?
Most people don’t realize that they’ve been living with beta emitters in their homes and offices. Smoke detectors use a beta emitter called americium, whose high-energy electrons are used to detect whether or not you have a fire. Other produced radioisotope devices are used in gun sights, and in signs for environments that cannot tolerate open flame or spark. The high-energy electrons hit a phosphor, which then glows.
What is the history of betavoltaic use in medical devices?
In the 1980s, people made betavoltaics out of promethium, another beta emitter, and these batteries were used to power pacemakers in patients. These pacemakers were actually implanted but had very limited distribution; there were about 400 or 500 of them. Promethium is a very high-energy beta producer, and when the electrons decelerate they produce x-rays that have to be shielded, which made the device more bulky.
How are tritium-based medical devices different from these older ones?
The energy of the electrons they emit is much, much lower, and they don’t produce any radiation, so the shielding requirements are almost nonexistent. Also the size of the pacemaker has shrunk and the amount of power it requires is now much less than it used to be. It’s a pretty good match to what a betavoltaic cell could produce. The lifetime of these betavoltaic cells is related to the half-life of the isotope, which is 12.5 years. (That is the period of time during which half of the radioisotope’s nuclear reactions, of hydrogen going to helium, have taken place.) Any kind of implantable device—such as one measuring pressure or perhaps temperature—could benefit, particularly when you need a very small form factor and power requirements are modest.
What is making betavoltaic devices attractive now?
What’s happening is that things are coming together. The electronics industry now knows how to make exquisitely low-power electronics. In addition, as in the case of the pacemaker, the footprint of devices has shrunk. Now it’s time for a reexamination of this technology, because the application space is coming to meet it. It has a small power output but an extremely high energy density.
How do these betavoltaic devices compare with traditional power sources such as batteries?
With normal batteries, like lithium ones, there are certain limits. As you make them smaller and smaller, it becomes more and more difficult to make them perform as well. On the other hand, a betavoltaic device naturally scales to the smaller dimensions. Lithium batteries are not very good when the temperature changes, something betavoltaics are in fact very resistant to.
The other thing that’s interesting to me is that we’re exploding into this idea of the Internet of things, but everybody is asking about the power sources. You can have a lithium battery, but it does not have a very long life, it’s pretty big, and it doesn’t work too well in relatively modest extreme environments. You can have a miniature solar cell, or a piezoelectric scavenger that will convert motion into energy, but if the Sun’s not shining and you’re not moving around, they don’t work.
My vision would be that you’d have a trilogy of sources, with the betavoltaic source being the one of last resort that would keep the computer processor alive. Then you would use the sources when they came online, when somebody turned the light on in the room, or you started moving and something started vibrating and you were able to get power that way. You start thinking of micropower as a microgrid with different sources being able to do the things that they do best.
Can betavoltaics devices also be used as batteries?
A betavoltaic is a power generator, not a storage device. But the power is always coming out, so you could capture it and store it in a capacitor or a thin-film battery. Then you could still have that power for another time.
Say you have an application where you wanted to monitor the temperature of an agricultural field over a long period of time. You’d put a lot of sensors down and have them take the temperature measurements. Making a record of that data doesn’t take much energy in today’s world; you can do that with a betavoltaic. But the readout, if you’re doing it with wi-fi, does take up a lot of energy. If you only wanted to read it out once every two weeks, you could probably store enough energy from the betavoltaic so that you could read out in a couple of minutes all of the data that you stored in two weeks of work. That’s a good application, because in this long-term monitoring, you’re looking for trends and not for actual data instantly.
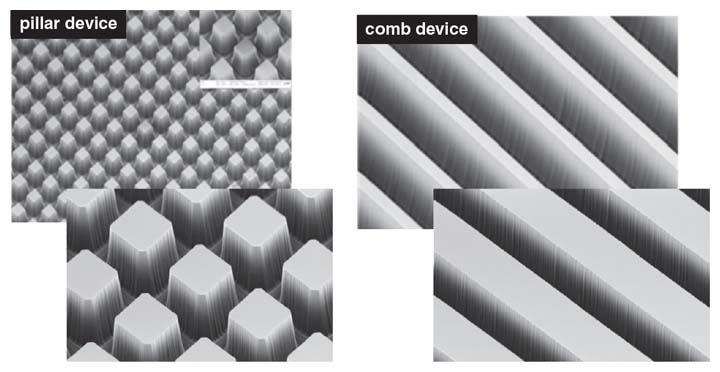
In your devices, the surface of the material is covered with tiny pillars (above). What advantage does that structuring give the betavoltaic?
Unlike solar cells, your energy source is right next to you, this titanium tritide. To increase the power for these betavoltaic devices, you create a larger surface area. Etching the surface puts more fuel on a given area of a device by a factor of a hundred, and in principle could increase the power by two orders of magnitude.
What are some additional potential applications for these betavoltaics?
Another interesting application is anti-tampering. Suppose you had a box of electronics that you didn't want people to look at. You need something that doesn’t have to have a lot of power but just has to be on all the time to detect a breakage when somebody opens it and then trigger an alarm. For that, a betavoltaic device is interesting.
If you wanted to store a cryptographic key electronically, again you want something that lives a long time with almost no power output. That’s a good application for a betavoltaic device as well.
When could these devices be commercially mass-produced?
I think you can get a working prototype for the medical area within one or two years and a production prototype within five years.
American Scientist Comments and Discussion
To discuss our articles or comment on them, please share them and tag American Scientist on social media platforms. Here are links to our profiles on Twitter, Facebook, and LinkedIn.
If we re-share your post, we will moderate comments/discussion following our comments policy.