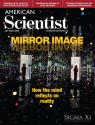
This Article From Issue
July-August 2019
Volume 107, Number 4
Page 206
Illustrator Kaija Saaremaeel is fascinated by the inner workings of plants, and has developed a regular series of plant morphology graphics.
Here she describes the life cycle of the tea plant, Camellia sinensis, from which a variety of teas are produced.
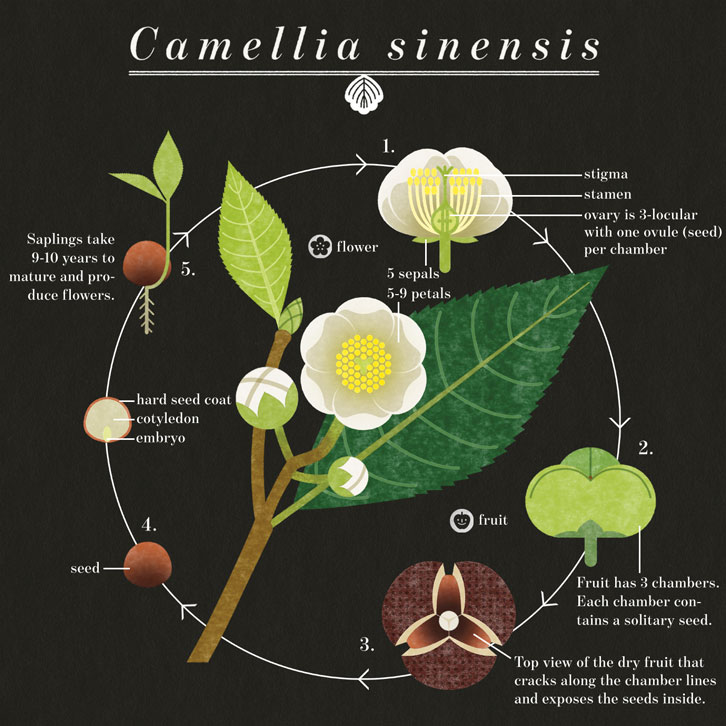
Infographic by Kaija Saaremaeel.
American Scientist Comments and Discussion
To discuss our articles or comment on them, please share them and tag American Scientist on social media platforms. Here are links to our profiles on Twitter, Facebook, and LinkedIn.
If we re-share your post, we will moderate comments/discussion following our comments policy.