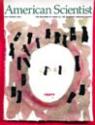
This Article From Issue
July-August 2003
Volume 91, Number 4
DOI: 10.1511/2003.26.0
Six Degrees: The Science of a Connected Age. Duncan J. Watts. 368 pp. W. W. Norton and Company, 2003. $27.95.
A successful new discipline in science is seldom recognized as such "in the first minute of creation"; only time can reveal whether it will have a lasting, pervasive influence. Yet a chorus of books (including recent titles by László Barabási and by Mark Buchanan) are now proclaiming, somewhat prematurely in my opinion, a "new science of networks." The latest of these—Six Degrees: The Science of a Connected Age, by Duncan Watts—offers the lay reader fascinating insights into the way science is done, particularly into the interplay of several fields (ranging from physics to sociology) with distinctive methodologies. Watts's account is personal, and compelling in its honesty.
He begins by observing that the classical theory of random networks has failed to explain the properties of many important entities, including social networks and the link structure of the World Wide Web. Watts argues that we need a new science that blends graph theory, stochastic processes and "complexity." Early in the book, he raises the issue of what happens in a large complex system of interconnected agents—an important general area—hinting that this is completely new ground. But in fact there are antecedents—for instance, in the theory of large deviations in queuing networks. Notwithstanding this, Watts adduces a number of convincing instances in which the simple models of classical random graph theory fail to explain, say, the structure of the Web. He develops a number of more sophisticated models that (in computer simulations) better mirror some empirical observations.
Along the way, he suggests that the classical theory of random graphs is a failure because for decades mathematicians overlooked the need to consider more sophisticated models. There is perhaps a simpler reason for this oversight: Mathematicians like to prove theorems about the objects they study, and when the objects and models get too complex for their proof techniques, they tend to back off. In contrast, almost all of the evidence driving the conclusions in Six Degrees rests on computer simulations, which allow the exploration of sophisticated network models without demanding definitive mathematical proof. Watts clearly recognizes this important difference (he makes this obvious in chapter five) but could have explained it better; however, the book otherwise nicely contrasts the scientific methodologies from multiple disciplines.
A number of interesting insights follow from this study in contrasts. For instance, late in chapter two Watts launches into what at first seems to be faint praise for physicists. Only after completely reading the chapter does one realize his deeper point—that what is obvious to scientists schooled in one discipline may initially be excruciatingly difficult for those in another. In other words, the way of thinking and the approach in different branches of sciences do not fall under a catchall "scientific method." In underscoring this, Watts makes a fundamental point about interdisciplinary science that deserves fuller appreciation not only by the lay reader but perhaps even by most scientists.
Watts skillfully varies his style of writing to strike a good balance between scientific accuracy and ease of reading. For instance, he nicely compares the Poisson and normal distributions as they pertain to the problems he describes, without leaving the reader awash in formulae. At the other end of the stylistic spectrum, he is able to pull off commencing a chapter with the sentence fragment "The island of Manhattan." It's immediately clear that something dramatically populist is about to follow.
Throughout, one gets insights into what drives scientists (there are, for instance, multiple accounts of priority and preemption in publication). Watts nevertheless succeeds in staying above the fray and is very generous in crediting the accomplishments of other authors.
Unfortunately, he occasionally ignores scientific reality in the interest of having the story read more smoothly. For example, the models of preferential attachment described for Web connectivity really did not turn out to explain the structure of the Web very well—the subject remains under active investigation.
To a far greater extent than the authors of earlier books on this subject, Watts does make an effort to stress that beyond the generality of these models, the details do matter. His account of the statistical and algorithmic significance of the classic experiments of social psychologist Stanley Milgram (which gave rise to the folklore that any two humans are separated by no more than six degrees of acquaintanceship) is especially striking, and the points Watts makes on the gap between what Milgram actually measured and what he is often construed to have measured should be more widely appreciated. The discussion of individual versus social good in economics is surprisingly light on the strong resurgence of interest in multiparty and computational game theory—one would certainly have expected at least a mention of John Nash or John von Neumann.
The material in the last three chapters seems poorly focused and appears to be an attempt to cover recent phenomena such as Napster, the aftermath of the September 11, 2001, attacks and the demise of the Long Term Capital Management hedge fund. Here the scientific focus that characterizes the earlier chapters dissipates into generalities.
Are Watts and his colleagues brilliantly prescient in auguring a "new science of networks," or have they jumped the gun? Only time will tell. But in either case, Six Degrees is a very entertaining read, especially illuminating for nonscientists.—Prabhakar Raghavan, Verity, Inc., Sunnyvale, California, and Computer Science, Stanford University
American Scientist Comments and Discussion
To discuss our articles or comment on them, please share them and tag American Scientist on social media platforms. Here are links to our profiles on Twitter, Facebook, and LinkedIn.
If we re-share your post, we will moderate comments/discussion following our comments policy.