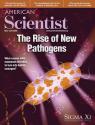
This Article From Issue
May-June 2022
Volume 110, Number 3
Page 186
THE ANNOTATED HODGKIN AND HUXLEY: A Reader’s Guide. Indira M. Raman and David L. Ferster. xiii + 311 pp. Princeton University Press, 2021. Cloth, $110; paper, $39.95.
The idea that nerves carry electrical signals between the brain and the body goes back to the 18th century, when Italian physician and physicist Luigi Galvani noticed that a small jolt of electricity would cause a dissected frog’s leg to twitch. But nerve fibers are not simple conductors like the copper wires of a telegraph network. Although electrical activity is key to nerve action, the process that moves a pulse of information along the fiber is a good deal more complicated than the flow of electrons through a wire.
The search for the true mechanism of nerve transmission culminated in a series of ingenious and painstaking experiments done by a pair of British physiologists: Alan Lloyd Hodgkin (1914–1998) and Andrew Fielding Huxley (1917–2012). They reported their results in five papers, which all appeared in the Journal of Physiology in 1952. Hodgkin and Huxley received a Nobel Prize for this work in 1963 (shared with John Eccles).
The five papers are printed in facsimile in The Annotated Hodgkin and Huxley, along with extensive commentary and background material supplied by the editors, Indira M. Raman and David L. Ferster, who are neurobiology professors at Northwestern University. The original papers are freely available on the Journal of Physiology website, but the Raman–Ferster annotations are a valuable resource.
Hodgkin and Huxley—or H&H, as they are called in the annotations—began their collaboration in the late 1930s. By then it was already apparent that nerves are not wires. The electric currents that constitute a nerve impulse do not flow along the length of a nerve; instead they are radial migrations of charged particles across the cell membrane, from inside to outside or vice versa. H&H set out to learn what sets these currents in motion, why they are extinguished after a few milliseconds, and how a localized electrical disturbance can propagate along the fiber as a wave of excitation, known as an action potential.
Their experiments required technical artistry. The first step was to extract from the body of a squid a few centimeters of a nerve fiber called the giant axon, the portion of the neuron that carries nerve impulses away from the cell body. (Actually, the very first step was to get a live squid, and the difficulty of doing so was a serious impediment at times.) Although the squid axon is “giant” compared with other nerve fibers, it’s typically only half a millimeter in diameter. So the next challenge was to insert into the interior of the axon a fine glass capillary tube with two even finer wire electrodes wrapped around it.
The two electrodes were used in a technique called a voltage clamp. One electrode served as a voltage sensor, measuring the electrical potential of the fluids inside the axon with respect to those outside. The signal from this sensor was delivered to the input of a feedback amplifier (an item of apparatus that H&H had to build for themselves). The output of the amplifier went to the second electrode, injecting current that opposed any change in the measured voltage. The aim of this arrangement was to hold the internal voltage constant—to “clamp” it—allowing changes in the behavior of the cell membrane to be measured with great precision.
The first four H&H papers describe several variations on this experiment, including a number of wrong turns, false leads, and dead ends, as well as ultimate success. The final paper presents the authors’ synthesis and interpretation of the results. Carefully marshaling evidence in support of their claims, they argue that an action potential begins with a rapid influx of sodium ions crossing the cell membrane from outside to inside. A counterflow of potassium ions leaving the cell has a slower onset but eventually becomes dominant. When equilibrium is restored, both flows stop. The delay between the sodium and potassium peaks creates a brief electrical imbalance, a voltage spike that we observe as an action potential.
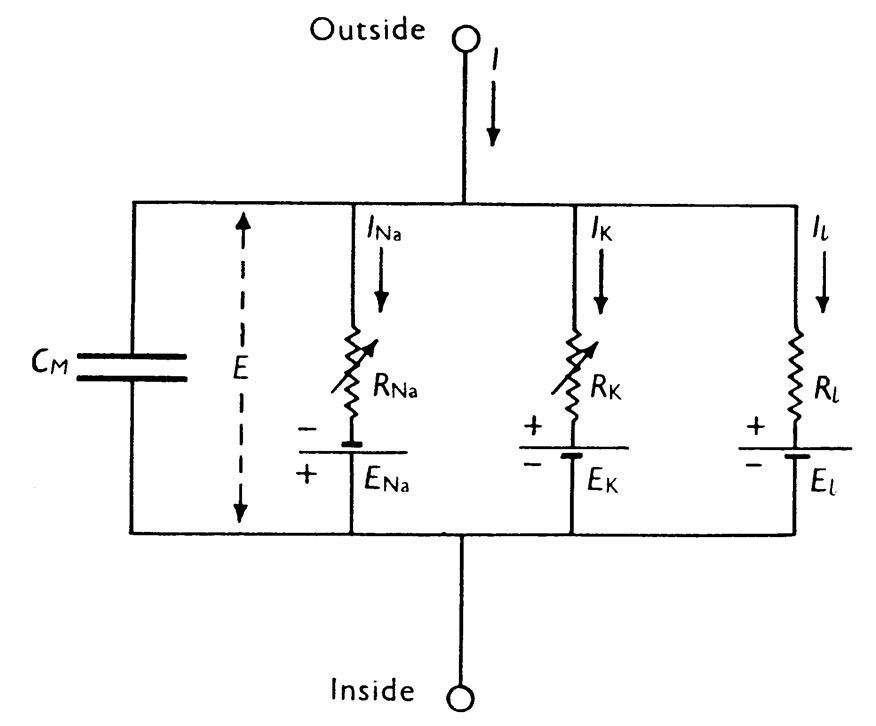
This diagram appeared in the last of five papers by Alan Hodgkin and Andrew Huxley published in 1952, titled “A Quantitative Description of Membrane Current and Its Application to Conduction and Excitation in Nerve.” Journal of Physiology 117:500–504. Reprinted by permission of the Physiological Society.
What stands out on first acquaintance with this body of work is that H&H are explaining biological phenomena in terms of ideas that would be more familiar to an electrical engineer. The foundation of their whole analysis is Ohm’s law: Current is equal to voltage divided by resistance. The first figure in the fifth paper (shown above) depicts the nerve-cell membrane as an assembly of resistors, capacitors, and such—parts one might solder onto a circuit board. Raman and Ferster comment: “The circuit diagram in Figure 1 is a landmark in the history of biophysics, illustrating how physical laws that govern nonliving material are also manifested in, and indeed give rise to, life processes.”
H&H go on from circuit diagrams to an even more abstract formalism: a set of equations that describe how the ionic currents rise and fall as a function of time and how they vary with distance along the length of the axon (see below). They inferred the equations from their experimental data, without the benefit of knowing anything about the underlying molecular structures responsible for the currents in the nerve cell. However, the formulation of the equations was not a matter of blind curve-fitting, where an equation is simply adjusted to minimize some measure of error. H&H were guided by their ideas about plausible biomolecular mechanisms, some of which turned out to be prescient. In particular, their model of the potassium current has a factor of n4, where n can vary from 0 to 1. The exponent 4 in this expression was selected because the potassium current’s slow onset suggested that four independent events are needed to initiate the flow. Two decades later it was discovered that the membrane protein serving as a potassium gate has four subunits, each of which must be activated to open the channel.
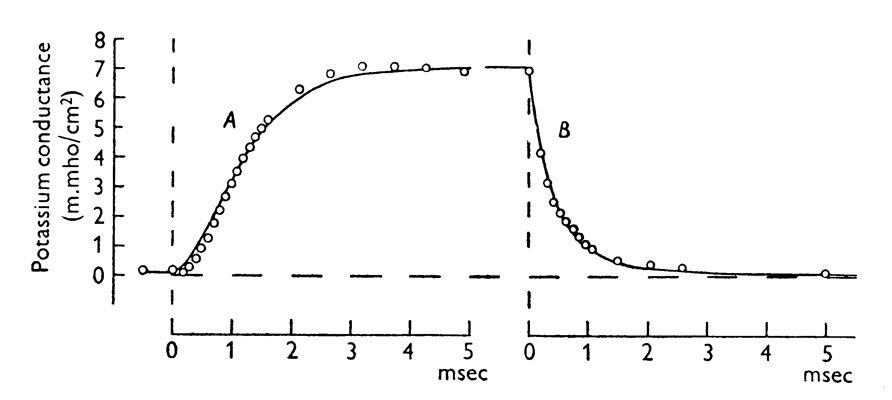
From Hodgkin, A. L., and A. F. Huxley. 1952. A quantitative description of membrane current and its application to conduction and excitation in nerve. Journal of Physiology 117:500–504. Reprinted by permission of the Physiological Society.
The full set of Hodgkin–Huxley equations has no exact solution. H&H had to work toward a solution using “numerical methods”; essentially, they made a guess and improved it by successive approximation. They had hoped to do this work on an electronic computer at the University of Cambridge (presumably the EDSAC, the Electronic Delay Storage Automatic Calculator, commissioned in 1949), but the machine was down for repairs when they needed it. Huxley did all the calculations with a hand-cranked mechanical calculator.
The work of Hodgkin and Huxley is described in countless textbooks, including some intended for introductory biology courses. Anyone with a serious interest in the neurosciences is sure to encounter these ideas at some point in their education. But I have the impression that few take the trouble to read the original sources. And why should they? Those papers from 1952 are badly out of date!
Raman and Ferster enumerate no fewer than seven reasons for revisiting these 70-year-old publications, but I think this one is sufficient: “The series of papers provide an exemplary (and arguably unparalleled) illustration of the scientific method at its best, with repeated sequences of observations, hypotheses, predictions, experimental tests, interpretations, evaluation of error, and consideration of plausible alternatives.” I would add that H&H also uphold a high standard of civility, viewing rivals and critics not as enemies to be vanquished but as colleagues in pursuit of a shared goal. This is happy science!
The Hodgkin–Huxley model of nerve transmission is a major landmark in the neurosciences, but it also has broader significance for biology as a whole. It is an important (and perhaps underappreciated) piece of the 20th-century movement that brought quantitative and analytical methods into a field that had been largely descriptive and observational.
Although Hodgkin and Huxley did many of their experiments at marine biology laboratories—that’s where the squid are—their home base was Trinity College, Cambridge. They were in good company there in the early 1950s. Just upriver from Trinity, at King’s College, James Watson and Francis Crick were puzzling over the structure of DNA. Across town at the Cavendish Laboratory, Max Perutz and John Kendrew were making progress on making sense of other macromolecules, such as hemoglobin. Most of these people were members of an informal Cambridge group called the Hardy Club, which had the explicit aim of bringing ideas from physics and mathematics into the life sciences. The chance to catch a whiff of the excitement of this extraordinary time and place is another reason to read the original papers rather than retrospective summaries.
The Raman–Ferster annotated edition makes an inviting package. At the front of the book the editors provide a historical introduction, beginning with Galvani and including some biographical notes on the principal figures in the story. Hodgkin, whose father had also been a student of natural sciences at Trinity, began with a naturalist’s interest in birds and botany, but he turned to research in physiology at age 20, while still an undergraduate. Huxley, who was a grandson of Thomas H. Huxley, the champion of Darwinian theory, arrived at Trinity planning to become an engineer, but in his third year he took a physiology course taught by Hodgkin and was soon engaged in the research program, also at age 20.
In the main part of the book, the text of the five H&H papers appears on left-hand pages, and the notes by Raman and Ferster appear on the facing right-hand pages. Many of the notes fill in background facts and assumptions that were better known in 1952 than they are now. Others bring the reader up to date on how ideas about nerve physiology have developed and changed in the decades since the papers were published. Still other notes point out elements of the work or the exposition that Raman and Ferster particularly admire.
Most of the comments seem to be addressed to biologists who might be flummoxed to encounter ideas from mathematics, physics, and electronic technology. I suppose those are the likeliest readers of the book. But I do wish the annotators had also pointed their spotlight into some of biology’s dark and dusty corners. I, for one, would have liked to learn how one goes about dissecting a squid and recovering, intact, that giant axon. I would also have welcomed a fuller discussion of all that has changed in neurophysiology since 1952. Mathematical models of nerve transmission are now supplemented by computational models, some of which are much more detailed; Raman and Ferster do mention one of these models, but only briefly.
Similarly, the five appendices that follow the book’s main text cover technical and mathematical aspects of the work that some biology students might find challenging. I would have liked to see an appendix on what’s known about the various ion channel proteins in neural membranes, and how they account for features of the Hodgkin–Huxley equations.
I must conclude with a confession. Although I have a long-standing interest in the neurosciences, and I’m a firm believer in seeking out primary sources, I had never read the Hodgkin–Huxley papers until this book came my way. I am grateful that it did.
American Scientist Comments and Discussion
To discuss our articles or comment on them, please share them and tag American Scientist on social media platforms. Here are links to our profiles on Twitter, Facebook, and LinkedIn.
If we re-share your post, we will moderate comments/discussion following our comments policy.